近几十年,地震学上一个非常重要的观测是在非洲和太平洋板块下方的深部地幔发现了两个大型剪切波低速体(large low shear velocity provinces, LLSVPs),它们在核幔边界上横向绵延几千公里,高数百公里,剪切波穿过时速度降低(He and Wen, 2012; Ni et al., 2002; Wang and Wen, 2007),造成这一现象的原因是该区域存在温度上的热异常甚至化学成分差异(Garnero et al., 2016)。
对于这两个LLSVPs的动力学演化过程,现有的研究分两类观点:
(1)有研究通过古地磁数据恢复320 Ma以来的大火成岩省和金伯利岩位置发现它们的位置几乎全部位于现今观测到的这两个大型剪切波低速体的内部或边缘(Torsvik et al., 2010; Torsvik et al., 2008),并据此推测大型剪切波低速体可能自320 Ma以来是相对固定不动的。基于物理模型的分析研究指出,离心力可能将大型剪切波低速体锁固在赤道附近的低纬度地区,最早追溯到2500 Ma(Dziewonski et al., 2010; Torsvik et al., 2014)。由于地磁场的轴对称性,古地磁数据可以恢复古板块的纬度信息,但是对于经度信息几乎无法确定。因此,如果大型剪切波低速体长期固定不动,那么则可以利用它们的这个特征建立参考系用以恢复古大陆的绝对位置。
(2)也有基于板块重建约束和自洽的地球动力学模型发现大型剪切波低速体很难在320 Ma以前保持固定不动(Langemeyer et al., 2020; Zhang et al., 2010; Zhong and Rudolph, 2015),并认为大型剪切波低速体与Pangea超大陆的形成相关,进一步提出大型剪切波低速体可能与超大陆旋回相关的模型(Li and Zhong, 2009)。虽然320 Ma以前,大型剪切波低速体是否固定不动存在着争议,但是有研究认为250 Ma以来的大型剪切波低速体是固定不动的(Conrad et al., 2013)。
针对以上问题,来自澳大利亚伍伦贡大学大学的Flament博士及其合作者通过1 Ga以来的板块重建结果约束的地球动力学模型,测试了不同地幔物性参数范围内的深部地幔结构(高密度的地球化学原始地幔物质源区,形成层析成像观测的大型剪切波低速体),并与恢复的火山喷发位置作对比,结果发现快速移动的深部地幔结构与火山喷发的位置存在着很深的联系,即火山喷发的位置都能落在深部地幔结构的边缘。这表明通过火山喷发的位置,无法确定大型剪切波低速体是否固定不动。这一研究结果,质疑了固定模型,为地球动力学模型符合古地磁观测结果提供了新的地球动力学机制,相关成果最近发表在Nature上。
该研究得到了与现今地震学层析成像观测的大型剪切波低速体一致的深部地幔结构,并且古地磁重建得到的火山喷发的位置都位于模型预测的深部地幔结构以及地震学观测的大型剪切波低速体的边缘。为了降低模型的不确定性,该研究还设计了22个地幔对流模型,测试不同板块重建模型、不同地幔物质属性的影响。模型结果显示,非洲下方深部地幔结构在160 Ma以前并不是连续的结构,在60 Ma形成连续的结构而非前人认为的200 Ma(图1e)(Young et al., 2019; Zhong and Rudolph, 2015)。在该模型中,大陆地壳物质主要在非洲地幔区域参与物质循环,而在太平洋地幔区域却很少发现大陆地壳物质,这与地球化学的观测一致(Doucet et al., 2020)。该研究进一步解释,地球化学观测到的非洲下方深部地幔结构含有大陆物质而太平洋下方却不含大陆物质,主要是由于过去1 Ga的非洲大陆半球的俯冲带更靠近大陆,因此下沉的俯冲板片可以将大陆物质带入到非洲下方的深部地幔结构中(图2)。

图1 320Ma以来下地幔结构与火山喷发位置(Flament et al., 2022)。灰色区域为低速区域。第一列为层析成像模型第二列为地幔对流模型;不同颜色的点代表不同年龄的火山喷发位置
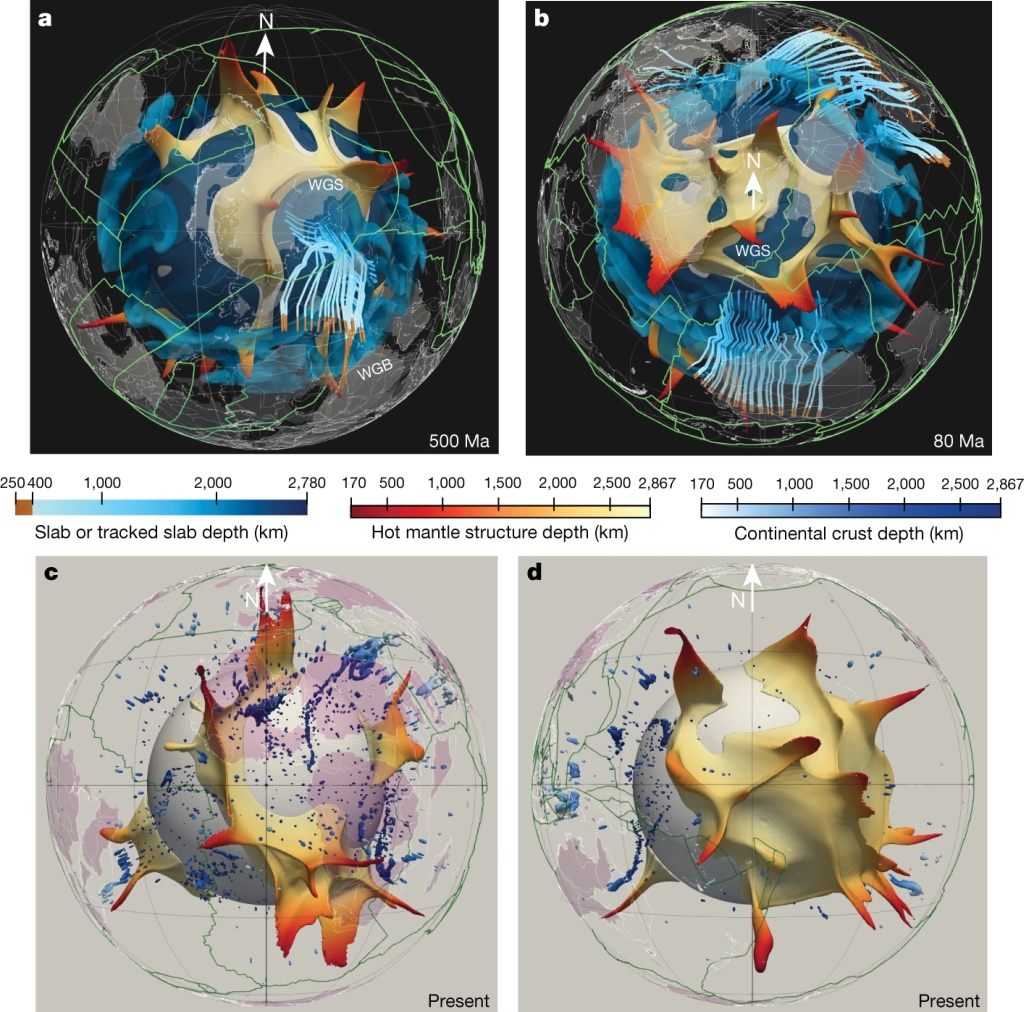
图2 非洲下方深部地幔结构演化与地幔中大陆地壳物质分布(Flament et al., 2022)
尽管该数值模型使用了目前最新也是最长的板块重构结果来约束模型的上表面边界状态,也可以很好的吻合部分观测结果,但也存在一些有待进一步改进的内容。在地表有1 Ga的板块重建结果约束,但是对于模型开始时(1 Ga)的深部地幔结构没有约束,因而假设1 Ga深部地幔结构是一层均匀的原始地幔物质组成。而当时的深部地幔是否存在着一层原始地幔物质层,以及原始地幔物质的形态都存在着诸多不确定性。如果1 Ga深部地幔结构不是均匀层状结构,而是已经形成了两个大型块体,则出现参考模型中效果所需要的深部地幔结构的密度就要更高,而密度的增大更会提高深部地幔结构的稳定性,从而影响模型预测的非洲下方LLSVP的聚合时间。另外,模型使用的板块重建结果是基于古地磁参考系的,并不是地幔坐标系。有研究发现,地幔参考系下的Pangea超大陆的位置、大小、形状与非洲下方大型剪切波低速体存在着更好的相似性(Mitchell et al., 2020)。因而使用真极移约束的地幔参考系的重建结果(Mitchell et al., 2012; Torsvik et al., 2014)而非研究使用的古地磁参考系(Merdith et al., 2021)的重建结果约束模型表面可能会使模型中的下地幔结构发生很大变化。有研究发现,增大热黏度差(相同压力条件下表面黏度与核幔边界黏度的比值)会增大深部地幔结构的稳定性(Li et al., 2014),而该研究使用的热黏度差偏小,如果使用较大的热黏度差,可能非洲下方的深部地幔结构更稳定。
无论如何,Flament等人的这项研究让我们得以从一个新的角度去继续探索和思考地球表面和深部结构之间的相互联系以及大型剪切波低速体在演化过程中到底是相对固定不动还是随着超大陆旋回快速移动这些关系到地球长期演化的基本问题。
主要参考文献
Conrad C P, Steinberger B, Torsvik T H. Stability of active mantle upwelling revealed by net characteristics of plate tectonics[J]. Nature, 2013, 498(7455): 479-482.
Doucet L S, Li Z X, Gamal El Dien H, et al. Distinct formation history for deep-mantle domains reflected in geochemical differences[J]. Nature Geoscience, 2020, 13(7): 511-515.
Dziewonski A M, Lekic V, Romanowicz B A. Mantle anchor structure: an argument for bottom up tectonics[J]. Earth and Planetary Science Letters, 2010, 299(1-2): 69-79.
Flament N, Bodur ? F, Williams S E, et al. Assembly of the basal mantle structure beneath Africa[J]. Nature, 2022, 603(7903): 846-851.(原文链接)
Garnero E J, McNamara A K, Shim S H. Continent-sized anomalous zones with low seismic velocity at the base of Earth's mantle[J]. Nature Geoscience, 2016, 9(7): 481-489.
He Y, Wen L. Geographic boundary of the “Pacific Anomaly” and its geometry and transitional structure in the north[J]. Journal of Geophysical Research: Solid Earth, 2012, 117(B9).
Langemeyer S M, Lowman J P, Tackley P J. The dynamics and impact of compositionally originating provinces in a mantle convection model featuring rheologically obtained plates[J]. Geophysical Journal International, 2020, 220(3): 1700-1716.
Li Y, Deschamps F, Tackley P J. The stability and structure of primordial reservoirs in the lower mantle: insights from models of thermochemical convection in three-dimensional spherical geometry[J]. Geophysical Journal International, 2014, 199(2): 914-930.
Li Z X, Zhong S. Supercontinent–superplume coupling, true polar wander and plume mobility: plate dominance in whole-mantle tectonics[J]. Physics of the Earth and Planetary Interiors, 2009, 176(3-4): 143-156.
Merdith A S, Williams S E, Collins A S, et al. Extending full-plate tectonic models into deep time: Linking the Neoproterozoic and the Phanerozoic[J]. Earth-Science Reviews, 2021, 214: 103477.
Mitchell R N, Kilian T M, Evans D A D. Supercontinent cycles and the calculation of absolute palaeolongitude in deep time[J]. Nature, 2012, 482(7384): 208-211.
Mitchell R N, Wu L, Murphy J B, et al. Trial by fire: Testing the paleolongitude of Pangea of competing reference frames with the African LLSVP[J]. Geoscience Frontiers, 2020, 11(4): 1253-1256.
Ni S, Tan E, Gurnis M, et al. Sharp sides to the African superplume[J]. Science, 2002, 296(5574): 1850-1852.
Torsvik T H, Burke K, Steinberger B, et al. Diamonds sampled by plumes from the core–mantle boundary[J]. Nature, 2010, 466(7304): 352-355.
Torsvik T H, Smethurst M A, Burke K, et al. Long term stability in deep mantle structure: Evidence from the~ 300 Ma Skagerrak-Centered Large Igneous Province (the SCLIP)[J]. Earth and Planetary Science Letters, 2008, 267(3-4): 444-452.
Torsvik T H, van der Voo R, Doubrovine P V, et al. Deep mantle structure as a reference frame for movements in and on the Earth[J]. Proceedings of the National Academy of Sciences, 2014, 111(24): 8735-8740.
Wang Y, Wen L. Geometry and P and S velocity structure of the “African Anomaly”[J]. Journal of Geophysical Research: Solid Earth, 2007, 112(B5).
Zhang N, Zhong S, Leng W, et al. A model for the evolution of the Earth's mantle structure since the Early Paleozoic[J]. Journal of Geophysical Research: Solid Earth, 2010, 115(B6).
Zhong S, Rudolph M L. On the temporal evolution of long‐wavelength mantle structure of the E arth since the early P aleozoic[J]. Geochemistry, Geophysics, Geosystems, 2015, 16(5): 1599-1615.
(撰稿:史志东,李杨/岩石圈室)